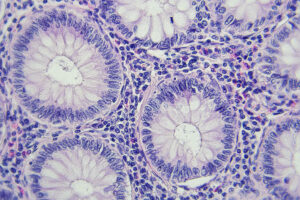
[Cancer image licensed from Adobe Stock]
Assessing CTC abundance and phenotypic changes, along with measuring ctDNA concentration, holds the potential for real-time tracking of disease progression, tumor burden and recurrence. Additionally, analyzing genetic and epigenetic alterations in CTCs and mutations in ctDNA over time, can offer valuable insights into the efficacy of therapeutic interventions, as the presence of different mutations could indicate cancer susceptibility or resistance to specific treatments (Figure 1). This dynamic approach has the potential to pave the way for a more personalized approach to cancer treatment.
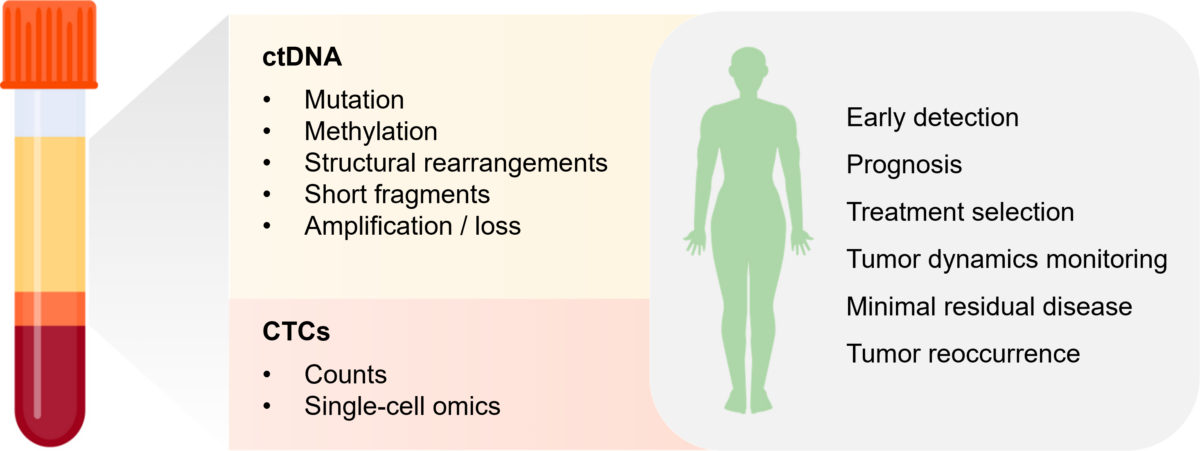
Figure 1. Example of circulating tumor material that could be isolated following a liquid biopsy and applications in monitoring cancer progression.
CTC capture-enrichment approaches
Advancements in CTC capture technologies are essential for integrating CTCs into clinical practice as a liquid biopsy analyte. To achieve this integration, CTC capture technologies must be unbiased, efficient, rapid, and cost-effective, consistently isolating a sufficient quantity of these cells for analysis. Moreover, these technologies should seamlessly integrate with advanced sequencing or quantitative tools and functional assays to provide data crucial for accurate therapeutic decision-making. However, the rarity and phenotypic diversity of CTCs pose significant challenges for cell enumeration and characterization, making CTC enrichment critical for reproducible downstream analysis.
Recent technological strides have led to the development of highly efficient and automatable techniques for capturing and enriching rare CTCs from liquid biopsy samples. Commercial instruments have emerged, employing both antigen-dependent and antigen-independent approaches. Antibody-based (i.e., antigen-dependent) CTC capture processes typically involve selective capture using biomarkers highly expressed on CTCs, such as the Epithelial Cell Adhesion (EpCAM) biomarker, often combined with negative selection of normal blood leukocytes cells using CD45-based methods to enhance assay specificity. However, CTCs transitioning to the mesenchymal state may exhibit low expression of these markers, which may result in them going undetected. Therefore, assays capable of detecting multiple biomarkers indicative of cancer progression and metastasis are crucial, with single-cell multi-omics analysis offering valuable insights when coupled with long-term follow-up data.
Antigen-agnostic detection technologies
In contrast, antigen-agnostic detection technologies leverage physical properties such as size, charge, density, or elasticity for CTC enrichment, overcoming challenges associated with biomarker expression. Methods include filter-based devices, density gradient centrifugation, capture surfaces, and microfluidic systems. Size-based selection, capturing cells in the CTC size range of 8-30µm, holds an advantage by not relying on biomarker expression, allowing for sorting and collection of CTCs with varying or alternative biomarker profiles.
Some innovative instruments offer dual functionality of CTC capture and immunostain-based enumeration in a single workflow, conducted on a microfluidic slide. Microfluidic platforms integrate biomarker-based positive and negative identification, imaging, and micromanipulation for the isolation of pure CTC subsets. Advances in capture technologies enable detailed molecular and functional analyses of CTCs at both bulk and single-cell levels, extending beyond simple enumeration. When combined with drug phenotyping, single-cell analysis of individual CTCs and CTC clusters can identify potential therapeutic targets. Although CTC capture and downstream analysis may exhibit clinical relevance, it is not yet routinely applied due to existing limitations. Addressing challenges related to CTC epitope expression, cell capture efficiencies, large blood volume processing, and workflow automation is imperative for widespread adoption in clinical settings.
ctDNA analysis using ddPCR and NGS technologies
In recent years, the field of cancer research has witnessed the rise of intricate molecular testing methods that offer deeper insights into tumor biological mechanisms. The combined use of Next-Generation Sequencing (NGS) and Droplet Digital PCR (ddPCR) has proven particularly advantageous in monitoring changes in ctDNA levels, detecting Minimal Residual Disease (MRD) (i.e. remaining cancer cells following treatment), and optimizing therapeutic strategies.
NGS and ddPCR, both highly capable technologies for liquid biopsy analysis, complement each other with their unique strengths. NGS excels at simultaneously evaluating unknown mutations across kilobases or megabases of a genome in a single run. However, NGS is labor-intensive, time-consuming, and prohibitively expensive for repeated, long-term monitoring applications. In addition, NGS results can vary from lab-to-lab, and data analysis is highly complex, usually requiring biostatistics expertise.
In contrast, ddPCR, based on nanoliter-sized water-in-oil emulsion droplet technology, is capable of absolute quantification of nucleic acids, including biomarkers of MRD and ctDNA. ddPCR is therefore well-suited for rare mutation detection, copy number variation analysis, DNA methylation analysis, and examination of gene rearrangements across various sample types (Figure 2). Compared to NGS, ddPCR is ideal for routine monitoring, offering same-day results, ease of set-up, high sensitivity, and precision at a relatively low cost, and offers straightforward, user-friendly analysis.
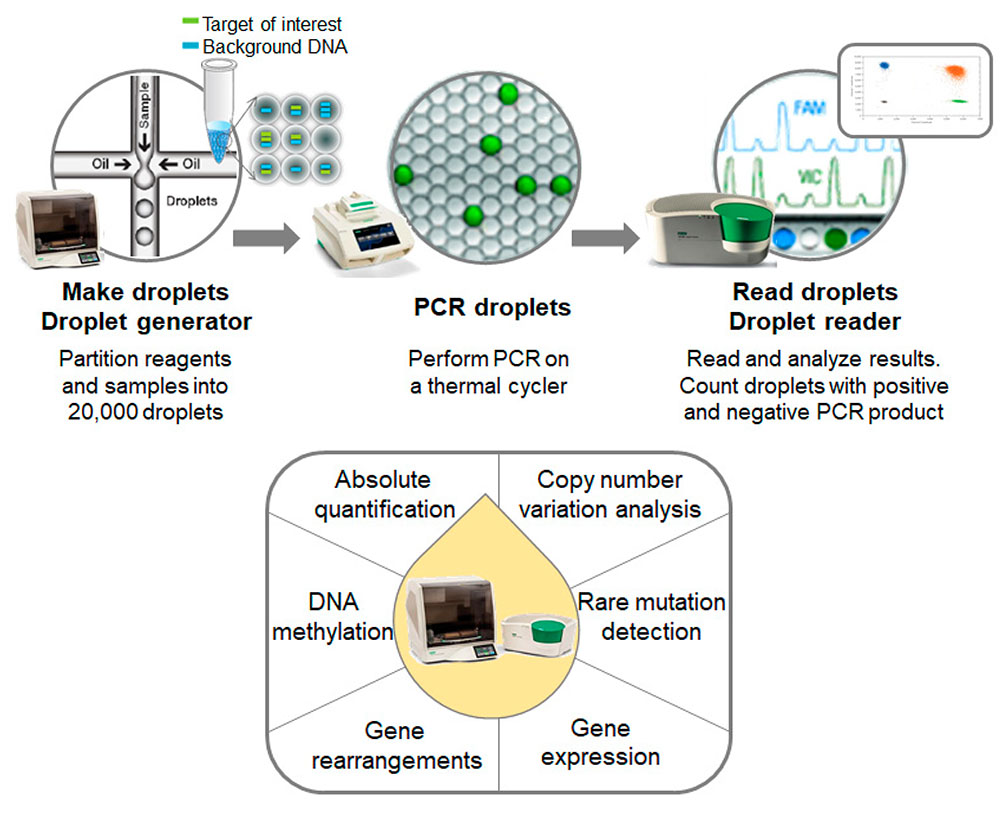
Figure 2. Overview of ctDNA screening process using ddPCR. Adopted from Huerta et al. 2021, under a CC BY 4.0.
The utility of ddPCR for mutation analysis research using liquid biopsy samples in various cancer types has been demonstrated by many studies. For example, ddPCR was successfully used to detect KRAS mutations in ctDNA of metastatic colorectal cancer samples, highlighting its capability to monitor therapy response. Likewise, this method has been instrumental in analyzing therapy response using samples from stage IV melanoma patients.
The promise of liquid biopsy techniques in cancer monitoring
Integrating data from both CTCs and ctDNA holds promise for a more comprehensive assessment of tumor aggressiveness, metastatic potential, and overall prognosis. Current research is directed towards addressing existing challenges in this field, with a focus on enhancing the specificity, reproducibility, and validation of detection systems based on ctDNA and CTCs. Simultaneously, advancements in microfluidics, artificial intelligence, and machine learning algorithms for data analysis, along with improvements in sequencing technologies, are being pursued to improve the accuracy and reliability of co-analysis. Effective collaboration among researchers, clinicians, and industry partners is crucial for the translation of these innovations into routine clinical practice.
Joby Chesnick, Ph.D., MBA is the senior global segment manager, single cell technologies, digital biology group, Bio-Rad Laboratories. Jeremiah McDole, Ph.D., oncology segment manager, digital biology group, Bio-Rad Laboratories.
Filed Under: Drug Discovery and Development, Genomics/Proteomics, Oncology